Year 4 Post-Doctoral Program (March 2023 - September 2024)
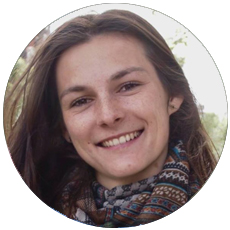
Dr. Solène Chiquier formerly gratudated from CentraleSupélec (Paris Saclay, France) and obtained a PhD on "The Implications of the Paris Agreement on Carbon Dioxide Removal (CDR) - Techno-Economics, Potential, Efficiency and Permanence of CDR pathways" at the Centre for Environmental Policy / Centre for Process Systems Engineering - Imperial College London in July 2022.
Very motivated by the NETs and CDR topics, she worked at the elaboration of a Research topic in coherence with the CarMa research objectives and based on the use of the MIT Emissions Production and Policy Analysis (EPPA) framework. In March 2023, she joined the CarMa Research Team while integrating the Sergey Paltsev's team at MIT.
The role and value of CDR pathways in delivering the Paris Agreement’s
1.5–2°C objectives
–
Integrating CDR within international/inter-regional carbon markets
–
International/Inter-regional Cooperation & Geo-Political Coalitions
Background
The need for CDR in delivering the Paris Agreement 1.5°C objectives
Technologies for carbon dioxide removal (CDR) from the atmosphere have been recognized as an important part of limiting warming to the well below 1.5–2°C called for in the 2015 Paris Agreement1,2. With global CO2 emissions still at high levels3, CDR technologies are becoming ever more important4–6. In particular, the absence of CDR would require unprecedented emissions reduction rates associated with high economic costs7,8, and the vast majority of the 2°C scenarios and all 1.5°C scenarios assessed in the Special Report on 1.5°C of the IPCC (SR15) required CDR to achieve the target1,9.
The techno-economic challenges of CDR
Various CDR options has been proposed in the literature — including afforestation/reforestation (AR)10, bioenergy with carbon capture and storage (BECCS)11, direct air capture with carbon capture and storage (DACCS)12,13, ocean fertilisation14, enhanced weathering (EW) of minerals15,16, biochar17,18 or soil carbon sequestration18,19. However, they have scarcely been taken up in Integrated Assessment Models (IAMs), often used to assess the techno-economic feasibility of delivering the Paris Agreement, notably in the IPCC SR15.
To that date, climate mitigation scenarios have included mainly AR and BECCS, DACCS as well, yet only recently, and seldom EW20–24, mainly because other CDR options remain speculative25. Particularly, the future role and value of each CDR technologies/practices remains unclear, owing to uncertainties relating potentials and costs26,27, and concerns about environmental sustainability26,28 but also relating to finance requirements29 and policy instruments30,31.
Particularly, only BECCS32 and DACCS33,34 have been deployed at the demonstration scale, yet nowhere near the scales required to deliver the Paris Agreement, and although AR is a well-established and mature practice, projects with the aim of removing CO2 from the atmosphere have only recently emerged (mostly in China35,36).
Due to the nascent nature of CDR options, concerns about the feasibility and sustainability of the largest-scale deployments CDR in 1.5°C-consistent scenarios have only been reinforced, especially if achieved via such limited portfolios of CDR options (i.e., only BECCS and AR)26–28,37.
Our proposal aims to address these knowledge gaps, by integrating a broader portfolio of CDR options in the EPPA/IGSM IAM developed at the MIT, Cambridge, USA. By explicitly accounting for region-specific bio-geophysical and socio-economic limits of AR, BECCS, biochar, DACCS and EW deployment, informed by underpinning physics-based models38, we aim to provide insights into the future feasible, sustainable and cost-effective deployment of CDR at scales consistent with the Paris Agreement’s 1.5–2°C objectives.
The socio-political and economic challenges of CDR
The absence of CDR targets in NDCs
As part of the Paris Agreement, Parties to the United Nations Framework Convention on Climate Change (UNFCCC) have to communicate/submit and update Nationally Determined Contributions (NDCs), in which they commit to pursue domestic climate mitigation measures, with the aim of achieving the Paris Agreement’s 1.5–2°C objectives. Since the close of COP26, many Parties, including the UK, the EU, Japan, and South Korea, have committed to legally-binding 2050 net-zero targets, while others, such as China and India, are set to do so by the second half of this century39,40. However, explicit CDR targets remain absent from all NDCs, with some exceptions related to the agriculture, and the land use, land-use change and forestry (LULUCF) sectors41,42.
The role of international/inter-regional carbon markets in pursuing NDCs
The Paris Agreement encourage Parties to pursue voluntary cooperation in the implementation of their NDCs “to allow for higher ambition in their mitigation and adaptation actions and to promote sustainable development and environmental integrity”. Particularly, Article 6 of the Paris Agreement introduces international policy instruments, such as Internationally Transferred Mitigation Outcomes (ITMOs) (i.e., transfers between domestic Emissions Trading Systems (ETS)), Voluntary Emission Reductions (VERs) (i.e., international market), or other non-market approaches, in which not only emissions reductions, but also negative emissions could be included. Deploying these international/inter-regional approaches is critical, as a) most nations are not on track with their 2030 and/or long-term NDCs43, and b) existing NDCs are insufficient to achieve most recent net zero commitments39,44.
Six years after the Paris Agreement, at COP26, Parties finally agreed and completed the rulebook of Article 6. However, it is still unknown how these market and non-market approaches could be implemented in the near-future. For context, domestic ETS, such as the EU ETS, the UK ETS or the California ETS are currently not linked to one another, i.e. there are no bilateral or multilateral transfers of carbon between them, as it is suggested the Paris Agreement’s ITMOs market-based approach.
Particularly, the integration of CDR technologies/practices remains challenging, owing to uncertainties relating (but not restricted to) the permanence, additivity and readily measurability and verifiability of CDR45,46, and to the financing of CDR45,47. For context, again, CDR options are not included yet in ETS.
The role of international cooperation in pursuing NDCs
The Paris Agreement aims to reflect equity and the principle of common but differentiated responsibilities and respective capabilities, in the light of different national circumstances. To do so, the Paris Agreement recognizes the need to support developing country Parties for its effective implementation, by encouraging all Parties to take into account the concerns of Parties with economies most affected by the impacts of response measures, particularly developing country Parties, when submitting their NDCs.
Whilst international cooperation has already been demonstrated to help delivering the Paris Agreement at scale, by allowing for cost-efficiency48, maintaining national sovereignty49, or reducing international inequalities24, the current increase of geo-political tensions poses a threat to its implementation, notably in the context of carbon markets or CDR. There might be a need to understand the impact of international/inter-regional cooperation, or geo-political coalitions, on the delivering of the Paris Agreement 1.5–2°C objectives.
Our proposal aims to address these knowledge gaps, by investigating the integration of CDR options into international/inter-regional markets for carbon, as suggested by Article 6 of the Paris Agreement, and thus their role in delivering net-zero targets, as submitted by the Parties in their NDCs. By explicitly accounting for region-specific socio-economic and geo-political factors within the EPPA/IGSM framework50, we aim to understand how international financial flows would need to be mobilize to be consistent with pathways towards low greenhouse gas emissions and climate-resilient development. Moreover, we also aim to provide insights into the role and value of international/inter-regional cooperation, and the impacts of geo-political coalitions, on future climate mitigation pathways.
Research hypothesis and objectives
On the basis of the foregoing text, we propose to 1) evaluate in this proposal the role and value of a broad portfolio of CDR technologies/practices, in line with the Paris Agreement’s 1.5–2°C objectives, 2) investigate their integration within international/inter-regional carbon markets, as suggested by Article 6 of the Paris Agreement, and 3) analyse the role and value of international/inter-regional cooperation, and the impact of geo-political coalitions on the delivering of the Paris Agreement’s 1.5–2°C objectives. The key questions which this proposal sets out to address are:
- What is the role and value of CDR in delivering the Paris Agreement’s 1.5–2°C objectives? How can a portfolio of CDR technologies/practices “fit” within the climate mitigation strategy?
- What is a feasible, sustainable, and region-specific portfolio of CDR technologies/practices, accounting for regional bio-geophysical resources and socio-economic factors?
- What is the role and value of international/inter-regional carbon markets, as suggested by Article 6 of the Paris Agreement? How can CDR be integrated in such markets?
- How do financial flows need to be mobilized to take most advantage of these market-based approaches, to deliver most cost-efficiently the Parties’ NDCs, whilst reflecting equity and the principle of common but differentiated responsibilities and respective capabilities?
- What is the role and value of international/inter-regional cooperation? What are the impacts of geo-political coalitions on the delivering of the Paris Agreement’s 1.5–2°C objectives and on the future development of regional economies?
To answer these questions, we have assembled a team of world class researchers from the leading academic and research institute Massachusetts Institute of Technology (MIT), Cambridge, USA. We plan to rely on their vast expertise for low-carbon energy systems transition and technology assessments.
Methodology
Our research programme comprises three interactive work packages (WPs), as shown in Figure 1. Together, these WPs will:
- Comparative Techno-Economic Assessment of CDR Options (WP1)
- Integration of CDR Deployment within Energy Systems and Land Use, and the Climate System (WP2)
- Assessment of International/Inter-Regional Carbon Markets, Cooperation and Geo-Political Coalitions (WP3)
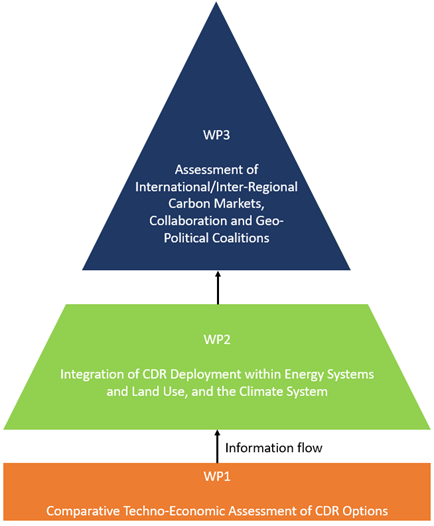
Figure 1. Work package structure with illustration of information flow between WPs
WP1: Comparative Techno-Economic Assessment of CDR Options
All CDR practices and technologies have the potential to generate negative CO2 emissions. However, they differ significantly in term of potential, cost, and side-effects, and are still subject to substantial technical and economic uncertainty. Particularly, BECCS is unique amongst CDR technologies in that it provides energy whilst removing CO2 from the atmosphere. But the production of biochar could also be optimised to generate bio-oil and heat in sufficient proportion to generate energy as well. DACCS, conversely, consumes significant amount of energy (heat and power), and if deployed at large-scale, could impact significantly the energy mix. AR, if deployed in a manner intended to, could provide ecosystems services by enhancing biodiversity and soil and water quality. Finally, biochar and EW could also enhance soil and nutrients quality and increase biomass yields. Overall, how should these assets best be utilised so as derive maximum value from them?
Aims: To provide a literature review of the potentials, costs and side-effects of a comprehensive portfolio of CDR options, and to provide insights into the key role that each of them will play in future net-zero energy systems, and society. Can they, and how, do they provide value? What are the Key Performance Indicators (KPIs) that determine affordability and sustainability of CDR options?
Deliverables: An academic review submitted to a peer reviewed journal.
WP2: Integration of CDR Deployment within Energy Systems and Land Use, and the Climate System
This WP evaluates the role of a range of CDR options at the global scale by integrating a portfolio of such CDR options (e.g., afforestation/reforestation, bioenergy with CCS (BECCS), direct air capture and carbon storage (DACCS), biochar, enhanced weathering (EW)) to the EPPA/IGSM framework. Key Performance Indicators (KPIs) characterising each element of the CDR portfolio, identified in WP1, will be quantified at the regional scale of the EPPA/IGSM framework*. WP2 will be intended to increase the technical resolution of the framework, therefore: 1) identifying the spatio-temporal emergence of a portfolio of CDR options, and 2) providing insights into potential synergies between CDR and other mitigation pathways (e.g., nuclear-DAC and sustainable aviation fuels (SAF)).
Aims: To expand the Economic Projection and Policy Analysis (EPPA) model to a broader portfolio of CDR technologies/practices using KPIs defined in WP1, In addition to EPPA, to use the Integrated Global System Modelling (IGSM) framework50 — an integrated assessment model (IAM), in which the interactions of human decision-making about energy systems and land use are simulated, at the regional and global scales, with the natural Earth system — to provide insights into the feasible and sustainable deployment of CDR within the climate mitigation strategy.
Task 2.1: To identify the CDR options that will be integrated within the EPPA/IGSM framework and quantify their KPIs (based on insights from WP1), at the spatial and temporal resolution of the EPPA/IGSM framework.
Task 2.2: To integrate the abovementioned KPIs within the EPPA/IGSM framework and to assess 1) the economic and technical value of a broad portfolio of CDR technologies/practices, and 2) potential synergies between CDR and other mitigation pathways, under different 1.5–2°C -consistent scenarios.
Deliverables: Academic paper under Task 2.1 and Task 2.2 submitted to a peer reviewed journal.
WP3: International/Inter-Regional Carbon Markets, Cooperation and Geo-Political Coalitions
Carbon markets are already playing a significant role into transitioning towards a net-zero economy by the second half of the century, by allowing nations to trade carbon credits to reduce their CO2 emissions. As suggested by Article 6 of the Paris Agreement, negative emissions are likely to be included in such carbon markets, at international/inter-regional scales. In order to realistically assess the viability of and effort level required to deploy CDR at the Paris Agreement’s scale (under different climate change mitigation scenarios), it is key to understand how CDR technologies/practices could be integrated within such carbon markets. Moreover, given the anticipated large scale of CDR required to be delivered to be consistent with the Paris Agreement’s 1.5–2°C objectives, it is also key to understand how valuable international/inter-regional cooperation would be in such carbon markets.
However, the threat of geo-political tensions has raised some concerns about future international/inter-regional cooperation, notably in the context of climate mitigation. Assuming that current political rhetoric and protectionist policies would lead to a decrease in international collaboration, this WP investigates different climate change mitigation scenarios, under which geo-political coalitions would be formed. Overall, such analysis will be essential to understand if, and how, different geo-political trajectories would improve or reduce 1) the feasibility and sustainability of the Paris Agreement’s 1.5–2°C objectives, and 2) regional inequalities, particularly between developed and developing country Parties.
Aims: To assess the techno-economic potential of CDR within international/inter-regional carbon markets, while delivering the Paris Agreement. To understand the implications of geo-political coalitions on the future deployment of climate mitigation scenarios, consistent with the Paris Agreement’s 1.5–2°C objectives, notably in terms of regional inequalities. Given outcomes of WP2, to understand how financial flows can be mobilized within international/inter-regional carbon markets most cost-efficiently (i.e., assuming international/inter-regional cooperation), or most conservatively/realistically (i.e., assuming geo-political coalitions) to deploy CDR technologies/practices within the global climate mitigation strategy.
International/Inter-Regional Carbon Markets and Cooperation
Task 3.1: To identify how CDR options can be integrated within international/inter-regional carbon markets, at the spatial and temporal resolution of the EPPA/IGSM framework, based on the existing literature. For example, to account for the timing, efficiency and permanence of different CDR options, e.g. risk of wildfires reducing AR’s CDR potential over time.
Task 3.2: To explore the implications of integrating CDR options within international/inter-regional carbon markets, particularly, in terms of financial flows. To contract the domestic NDCs submitted by each Party to the UNFCCC, and the Paris Agreement’s principle of common but differentiated responsibilities and respective capabilities, and to determine most cost-efficient climate mitigation scenarios, owing to international/inter-regional cooperation.
International/Inter-Regional Geo-Political Coalitions
Task 3.3: To identify how geo-political coalitions could be characterised in the EPPA/IGSM framework, i.e. how they could translate into constraints to the deployment of CDR options, as well as other climate mitigation options.
Task 3.4: To investigate the impact of geo-political coalitions within international/inter-regional carbon markets on the deployment of CDR and other climate mitigation options. To evaluate the socio-economic consequences of such coalitions at the regional scale.
Deliverables: Academic paper under Task 3.2 and Task 3.4 submitted to a peer reviewed journal.
Associated Resources
We propose the research project duration of 1 year ½ (See Figure 2).
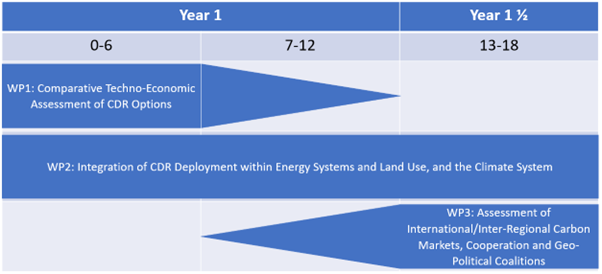
Figure 2. Project work plan
Track Records & Capacity Building
To deliver this ambitious and novel project, we propose a research project based at the Massachusetts Institute of Technology (MIT), in collaboration with the CarMa Chair.
The project will be supervised by Sergey Paltsev, Senior Research Scientist at MIT, and carried out by Solene Chiquier, a Postdoctoral Researcher based at MIT, who will focus on learning, implementing, and analysing CDR in the EPPA/IGSM framework.
Sergey Paltsev is a Deputy Director of the MIT Joint Program on the Science and Policy of Global Change, a Senior Research Scientist at the MIT Energy Initiative and MIT Center for Energy and Environmental Policy Research (CEEPR), and a Director of the MIT Energy-at-Scale Center, MIT, Cambridge, USA. He is the lead modeler in charge of the MIT Economic Projection and Policy Analysis (EPPA) model of the world economy, integrated within the MIT Integrated Global System Modeling (IGSM) model. His research covers a wide range of topics including energy economics, climate policy, taxation, advanced energy technologies, and international trade. Sergey is an Advisory Board Member for the Global Trade Analysis Project (GTAP) Consortium and a Member of the Economy-Wide Modeling Panel for the US Environmental Protection Agency (EPA) Science Advisory Board. He published more than 100 peer-reviewed publications in scientific journals and books. Finally, Sergey was a Lead Author of the Fifth Assessment Report (AR5) of the Intergovernmental Panel on Climate Change (IPCC).
Solene Chiquier is a PhD Student (expected date of completion is September 2022), and a Research Assistant at the Centre for Environmental Policy and at the Centre for Environmental Policy and the Centre for Process Systems Engineering at ICL. Her research covers the techno-economic potential of carbon dioxide removal (CDR) technologies, as well as their impacts on other sectors of the society (e.g., land, water, energy, jobs). Solene is the lead Researcher on Work Package 7 of the European Project NEGEM, a Research and Innovation Action funded by the EU Horizon 2020 Programme, in which she is developing an optimisation tool for assessing the realistic potential of CDR technologies in the European Union and their contribution to climate neutrality, as a supplementary strategy to emissions mitigation. Finally, Solene published 4 papers (3 under review), and 1 book chapter.
References
1 Intergovernmental Panel on Climate Change, Global Warming of 1.5°C. An IPCC Special Report on the impacts of global warming of 1.5°C above pre-industrial levels and related global greenhouse gas emission pathways, in the context of strengthening the global response to the threat of climate change, Press, 2018.
2 Intergovernmental Panel on Climate Change, Climate Change 2021: The Physical Science Basis. Contribution of Working Group I to the Sixth Assessment Report of the Intergovernmental Panel on Climate Change, Cambridge., 2021.
3 J. Friedlingstein, P., Jones, M. W., O’Sullivan, M., Andrew, R. M., Bakker, D. C. E., Hauck, J., Le Quéré, C., Peters, G. P., Peters, W., Pongratz, J., Sitch, S., Canadell, J. G., Ciais, P., Jackson, R. B., Alin, S. R., Anthoni, P., Bates, N. R., Becker, M., Earth Syst. Sci. Data Discuss., , DOI:110.5194/essd-2021-386.
4 G. Luderer, C. Bertram, K. Calvin, E. De Cian and E. Kriegler, Clim. Change, 2016, 136, 127–140.
5 B. M. Sanderson, B. C. O’Neill and C. Tebaldi, Geophys. Res. Lett., 2016, 43, 7133–7142.
6 B. M. Sanderson and B. C. O’Neill, Sci. Rep., 2020, 10, 1–12.
7 J. Strefler, N. Bauer, E. Kriegler, A. Popp, A. Giannousakis and O. Edenhofer, Environ. Res. Lett., 2018, 13, 044015.
8 E. Kriegler, G. Luderer, N. Bauer, L. Baumstark, S. Fujimori, A. Popp, J. Rogelj, J. Strefer and D. P. Van Vuuren, Philos. Trans. R. Soc. A Math. Phys. Eng. Sci., , DOI:10.1098/rsta.2016.0457.
9 J. Rogelj, A. Popp, K. V. Calvin, G. Luderer, J. Emmerling, D. Gernaat, S. Fujimori, J. Strefler, T. Hasegawa, G. Marangoni, V. Krey, E. Kriegler, K. Riahi, D. P. Van Vuuren, J. Doelman, L. Drouet, J. Edmonds, O. Fricko, M. Harmsen, P. Havlík, F. Humpenöder, E. Stehfest and M. Tavoni, Nat. Clim. Chang., 2018, 8, 325–332.
10 J. G. Canadell and M. R. Raupach, Science (80-. )., 2008, 320, 1456–1457.
11 M. Obersteiner, C. Azar, S. Kossmeier, R. Mechler, K. Möllersten, S. Nilsson, P. Read, Y. Yamagata and J. Yan, Managing Climate Risk, Austria, 2001.
12 R. Socolow, M. Desmond, R. Aines, J. Blackstock, O. Bolland, T. Kaarsberg, N. Lewis, M. Mazzotti, A. Pfeffer, K. Sawyer, J. Siirola, B. Smit and J. Wilcox, Direct Air Capture of CO2 with Chemicals: A Technology Assessment for the APS Panel on Public Affairs, 2011.
13 D. W. Keith, G. Holmes, D. St. Angelo and K. Heidel, Joule, 2018, 2, 1573–1594.
14 P. Renforth and T. Kruger, Energy and Fuels, 2013, 27, 4199–4207.
15 T. M. Lenton and C. Britton, Global Biogeochem. Cycles, , DOI:10.1029/2005GB002678.
16 R. D. Schuiling and P. Krijgsman, Clim. Change, 2006, 74, 349–354.
17 D. Woolf, J. E. Amonette, F. A. Street-Perrott, J. Lehmann and S. Joseph, Nat. Commun., , DOI:10.1038/ncomms1053.
18 P. Smith, Glob. Chang. Biol., 2016, 22, 1315–1324.
19 B. Minasny, B. P. Malone, A. B. McBratney, D. A. Angers, D. Arrouays, A. Chambers, V. Chaplot, Z. S. Chen, K. Cheng, B. S. Das, D. J. Field, A. Gimona, C. B. Hedley, S. Y. Hong, B. Mandal, B. P. Marchant, M. Martin, B. G. McConkey, V. L. Mulder, S. O’Rourke, A. C. Richer-de-Forges, I. Odeh, J. Padarian, K. Paustian, G. Pan, L. Poggio, I. Savin, V. Stolbovoy, U. Stockmann, Y. Sulaeman, C. C. Tsui, T. G. Vågen, B. van Wesemael and L. Winowiecki, Geoderma, 2017, 292, 59–86.
20 A. Marcucci, S. Kypreos and E. Panos, Clim. Change, 2017, 144, 181–193.
21 J. Strefler, N. Bauer, E. Kriegler, A. Popp, A. Giannousakis and O. Edenhofer, Environ. Res. Lett., 2018, 13, 044015.
22 G. Realmonte, L. Drouet, A. Gambhir, J. Glynn, A. Hawkes, A. C. Köberle and M. Tavoni, Nat. Commun., 2019, 10, 1–12.
23 J. Fuhrman, H. McJeon, P. Patel, S. C. Doney, W. M. Shobe and A. F. Clarens, Nat. Clim. Chang., 2020, 10, 920–927.
24 J. Strefler, N. Bauer, F. Humpenöder, D. Klein, A. Popp and E. Kriegler, Environ. Res. Lett., 2021, 16, 074021.
25 J. Fuhrman, H. McJeon, S. C. Doney, W. Shobe and A. F. Clarens, Front. Clim., 2019, 1, 11.
26 S. Fuss, W. F. Lamb, M. W. Callaghan, J. Hilaire, F. Creutzig, T. Amann, T. Beringer, W. De Oliveira Garcia, J. Hartmann, T. Khanna, G. Luderer, G. F. Nemet, J. Rogelj, P. Smith, J. V. Vicente, J. Wilcox, M. Del Mar Zamora Dominguez and J. C. Minx, Environ. Res. Lett., 2018, 13, 063002.
27 G. F. Nemet, M. W. Callaghan, F. Creutzig, S. Fuss, J. Hartmann, J. Hilaire, W. F. Lamb, J. C. Minx, S. Rogers and P. Smith, Environ. Res. Lett., 2018, 13, 063003.
28 P. Smith, S. J. Davis, F. Creutzig, S. Fuss, J. Minx, B. Gabrielle, E. Kato, R. B. Jackson, A. Cowie, E. Kriegler, D. P. Van Vuuren, J. Rogelj, P. Ciais, J. Milne, J. G. Canadell, D. McCollum, G. Peters, R. Andrew, V. Krey, G. Shrestha, P. Friedlingstein, T. Gasser, A. Grübler, W. K. Heidug, M. Jonas, C. D. Jones, F. Kraxner, E. Littleton, J. Lowe, J. R. Moreira, N. Nakicenovic, M. Obersteiner, A. Patwardhan, M. Rogner, E. Rubin, A. Sharifi, A. Torvanger, Y. Yamagata, J. Edmonds and C. Yongsung, Nat. Clim. Chang., 2016, 6, 42–50.
29 J. Bednar, M. Obersteiner and F. Wagner, Nat. Commun., 2019, 10, 1–4.
30 M. Honegger and D. Reiner, Clim. Policy, 2018, 18, 306–321.
31 R. Bellamy, Nat. Energy, 2018, 3, 532–534.
32 Drax Group Plc, https://www.drax.com/about-us/our-projects/bioenergy-carbon-capture-use-and-storage-beccs/, (accessed 9 December 2020).
33 Carbon Engineering Ltd, https://carbonengineering.com/our-technology/, (accessed 9 December 2020).
34 Climeworks, Climeworks, https://www.climeworks.com/co2-removal, (accessed 9 December 2020).
35 W. Bank, China: Afforestation Project in Shandong Improves Environment and Farmers’ Incomes, https://www.worldbank.org/en/results/2017/07/26/china-afforestation-project-in-shandong-improves-environment-and-farmers-incomes, (accessed 25 May 2021).
36 NASA LCLUC, Afforestation Along the Gobi Desert’s Margin, Northern China, https://lcluc.umd.edu/hotspot/afforestation-along-gobi-deserts-margin-northern-china-0, (accessed 25 May 2021).
37 V. Heck, D. Gerten, W. Lucht and A. Popp, Nat. Clim. Chang., 2018, 8, 151–155.
38 S. Chiquier, M. Fajardy and N. Mac Dowell, arXiv, , DOI:10.48550/arxiv.2204.04029.
39 Climate Analytics and New Climate Institute, Glasgow’s 2030 credibility gap: net zero’s lip service to climate action - Warming Projections Global Update, 2021.
40 United Nations Framework Convention on Climate Change (UNFCCC), Nationally determined contributions under the Paris Agreement, Glasgow, 2021.
41 S. L. Lewis, C. E. Wheeler, E. T. A. Mitchard and A. Koch, Nat. 2021 5687750, 2019, 568, 25–28.
42 L. and D. (FELD) A. T. The Food and Land Use (FOLU) Coalition and the Food, Environment, From Global Commitments to National Action: A Closer Look at Nationally Determined Contributions from a Food and Land Perspective, 2021.
43 Climate Analytics and New Climate Institute, Countries | Climate Action Tracker, https://climateactiontracker.org/countries/, (accessed 10 February 2022).
44 A. Geiges, A. Nauels, P. Yanguas Parra, M. Andrijevic, W. Hare, P. Pfleiderer, M. Schaeffer and C. F. Schleussner, Earth Syst. Dyn., 2020, 11, 697–708.
45 M. J. Mace, C. L. Fyson, M. Schaeffer and W. L. Hare, Glob. Policy, 2021, 12, 67–81.
46 M. Brander, F. Ascui, V. Scott and S. Tett, Clim. Policy, 2021, 21, 699–717.
47 M. Honegger, M. Poralla, A. Michaelowa and H.-M. Ahonen, Front. Clim., 2021, 3, 50.
48 M. Fajardy and N. Mac Dowell, One Earth, 2020, 3, 214–225.
49 N. Bauer, C. Bertram, A. Schultes, D. Klein, G. Luderer, E. Kriegler, A. Popp and O. Edenhofer, Nature, 2020, 588, 261–266.
50 J. Morris, A. Sokolov, A. Libardoni, C. Forest, S. Paltsev, J. Reilly, A. Schlosser, R. Prinn, H. Jacoby, J. Morris, A. Sokolov, A. Libardoni, C. Forest, S. Paltsev, J. Reilly and A. Schlosser, Report 349: A Consistent Framework for Uncertainty in Coupled Human-Earth System Models, 2021.
* The EPPA-IGSM framework models the world into 18 regions.
![]() |
Integrated assessment of carbon dioxide removal portfolios: land, energy, and economic trade-offs for climate policy Chiquier, Solene; Gurgel, Angelo; Morris, Jennifer; Chen, Yen-Heng Henry; Paltsev, Sergey (2025). In : Environmental Research Letters, vol. 20, n° 2, p. 24002. DOI: 10.1088/1748-9326/ada4c0. |
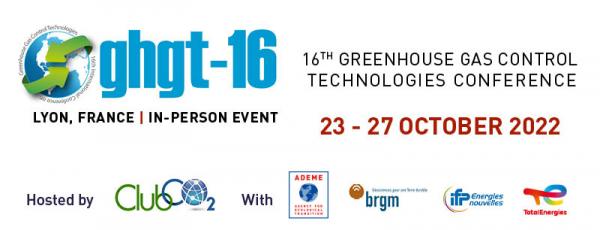
CarMa at GHGT16 next October in Lyon, France
The CarMa research team will be present at GHGT16 with up to 4 communications, with one in partnership with our colleagues from Imperial College London.